Mining and Refining: The Halogens
I was looking at the periodic table of the elements the other day, as one does, when my eye fell upon the right-hand side of the chart. Right next to …read more
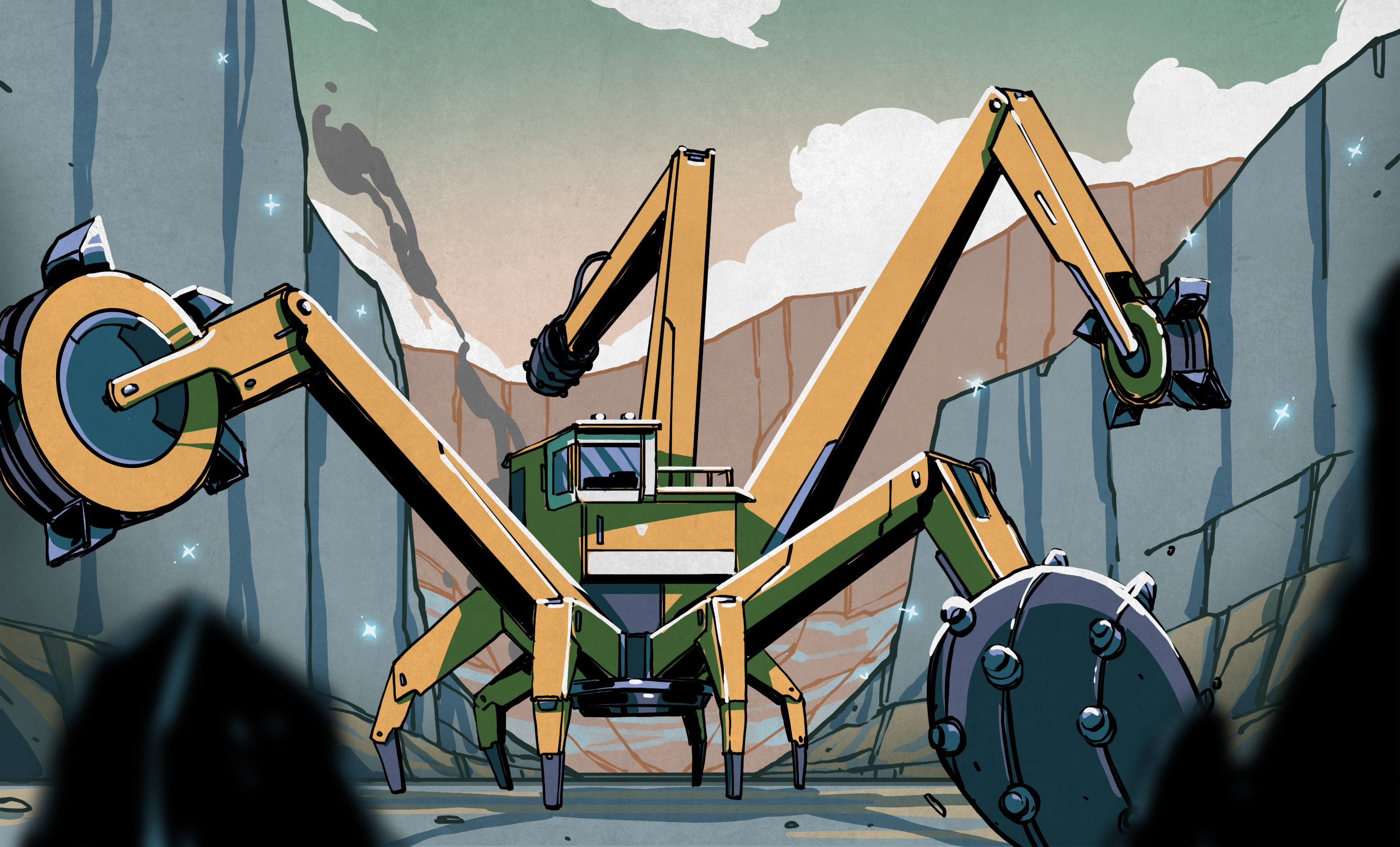
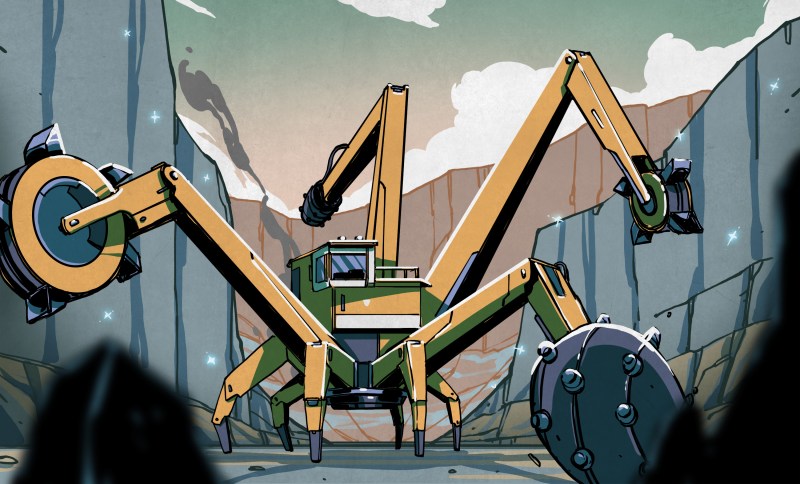
I was looking at the periodic table of the elements the other day, as one does, when my eye fell upon the right-hand side of the chart. Right next to the noble gases at the extreme edge of the table is a column of elements with similar and interesting properties: the halogens. Almost all of these reactive elements are pretty familiar, especially chlorine, which most of us eat by the gram every day in the form of table salt. As the neighborhoods of the periodic table go, Group 17 is pretty familiar territory.
But for some reason, one member of this group caught my attention: iodine. I realized I had no idea where we get iodine, which led to the realization that apart from chlorine, I really didn’t know where any of the halogens came from. And as usual, that meant I needed to dig in and learn a little bit about the mining and refining of the halogens. At least most of them; as interesting as they may be, we’ll be skipping the naturally occurring but rare and highly radioactive halogen astatine, as well as the synthetic halogen tennessine, which lives just below it in the group.
Fluorine
We’ll start our look at the halogens with fluorine, partly because we’ve already covered the production of hydrofluoric acid, the primary industrial source of elemental fluorine, but also because it’s a bit of an outlier compared to the other halogens, all of which are most commonly sourced from brines. Rather, hydrofluoric acid comes from rocks, specifically fluorspar, which produces HF and calcium sulfate when treated with sulfuric acid.
Hydrofluoric acid is converted into fluorine gas by electrolysis. Unfortunately, HF is a poor electrical conductor, so some potassium bifluoride is added to make the solution conductive enough for electrolysis. No water is used to make the electrolyte; rather, the HF and potassium bifluoride mixture is kept molten at up to 250°C in the electrolysis cell. HF is continuously added as electrolysis proceeds, to keep the electrolyte at the correct ratio. The steel walls of the cell act as the cathode, while a block of graphite is used as the anode. Fluorine gas is captured from the anode, dried and filtered, and compressed for storage.
Most industrial processes requiring fluorine get it from hydrofluoric acid, so only about 2% of all fluorine mined as fluorspar makes it into fluorine gas. The primary use for fluorine gas is the production of uranium hexafluoride, the feedstock for uranium purification for nuclear fuels. Most of the rest of fluorine gas production goes to the manufacture of sulfur hexafluoride for the electrical industry, where it serves as a gaseous dielectric for high-voltage switchgear.
Chlorine
Next up on our tour of the halogens is chlorine, a reactive element with so many industrial uses it’s hard to name them all. Apart from its familiar uses in disinfection and public sanitation, chlorine is used to create polymers like polyvinyl chloride along with a host of organic and inorganic chlorides. Chlorine is also needed to produce the next halogen on our list, bromine, which we’ll cover below.
Like fluorine gas, gaseous chlorine is produced by electrolysis using the chlor-alkali process. The electrolyte for chlorine production, though, is an aqueous brine solution, normally sourced from naturally occurring deposits that form by groundwater seeping into underground salt formations. The concentrated NaCl solution — or sometimes potassium chloride; the method works for both — is pumped to the surface from great depths and filtered to high purity before being piped into the cell house, where long rows of special electrolysis cells are ready to receive it.
Each electrolysis cell is divided into two compartments, one for the anode and one for the cathode. Between them is a thin membrane made from a resin material that is selectively permeable to cations. Fresh brine is pumped into the anode side of the cell, while plain water is pumped into the cathode side. On the anode side, the chlorine gas is liberated from the chloride ions and collected. The positively charged sodium or potassium ions move across the membrane into the cathode side of the cell. There, water is electrolyzed to hydrogen gas, a valuable byproduct that is also collected and, and hydroxide ions, which greedily bind with the sodium or potassium from the other side of the cell. This creates the third useful product of the chlor-alkali process, either sodium hydroxide or potassium hydroxide, which is generically referred to as caustic. The caustic is pumped off, filtered, and further purified before being shipped.
The raw chlorine gas coming off the anode side of the cell has a lot of water vapor mixed in with it. The gas is dried by passing it through a heat exchanger that cools it enough to condense the water vapor. The dried gas is then further cooled and compressed before being liquified for shipping. Alternatively, the dried gas can be piped to other parts of the plant for immediate use in other processes, including the production of bromine.
Bromine
In elemental terms, bromine is a strange beast. It’s the only non-metallic element that exists as a liquid under standard temperature and pressure conditions. That presents both challenges and opportunities for its extraction and purification.
As with most halogens, brine is the source material for bromine production. Sodium chloride is the main salt in most brines, but in places such as the Dead Sea area on the border between Israel and Jordan, the brine has enough bromides to be commercially viable. The Smackover Formation, which stretches in an arc from Texas to Florida in the United States also has bromide-rich brines, particularly in southern Arkansas.
Extraction starts with pumping brine up from deep wells. The brine is naturally hot, which reduces the amount of energy needed to extract the bromine. Filtered brine is pumped to the top of a tall reaction tower through a long vertical section of pipe. At the bottom of the pipe, chlorine gas is injected into the brine. Chlorine, a powerful oxidant, preferentially strips electrons away from bromides in the brine, which converts bromides to bromine (Br2). The brine enters the top of the reactor tower, which has an outlet at the top that’s connected to a venturi. High-pressure steam passes over the venturi, creating a partial vacuum in the tower. The combination of heat and vacuum causes the bromine in the brine to flash-evaporate. The gaseous bromine is sucked out of the venturi into the steam, while the remaining brine falls back down to the bottom of the tower through a matrix of plastic discs. The discs break the droplets up and slow them down, giving any remaining bromine in the brine a chance to evaporate and make its way back up and out of the tower.
The bromine gas is sent to a condenser to remove water vapor and another, colder condenser to turn it into a liquid. The liquid bromine then goes into a dryer that removes all remaining traces of water, chlorine, and other contaminants. The liquid bromine is then shipped in specialized rail or truck tankers while waste brine is pumped back into the geologic formation it came from. Bromine has many industrial uses, but its most familiar use is probably in flame-retardent compounds. Most of us have probably seen the sickly orange color of old plastics, which is caused by the bromine-based flame retardants breaking down over time. Bromine compounds also figure prominently in the FR4 substrate used to make flame-retardant printed circuit boards.
Iodine
Last up is iodine, the halogen that kicked this whole thing off. Iodine is unique in that it used to be extracted mainly from plants, specifically kelp. The discovery of a type of soil called caliche in the Atacama Desert of Chile changed things in the 19th century. While mainly prized as a rich source of potassium nitrate, some caliche deposits also have iodate minerals, up to 1% by weight. It’s not much, but it’s enough to make extraction commercially viable.
The current process for extracting iodine starts with making an artificial brine. Caliche is blasted free from the ground and piled into huge plastic-lined pits. Water is pumped over the caliche, which leaches the iodates as it works its way down through the pile. The resulting brine is pumped into evaporation ponds, where solids settle out of solution as the brine concentrates in the fierce desert sun.
The concentrated brine is pumped out of the ponds and filtered before being sent to large absorption towers. There, sulfur dioxide gas is injected into the brine, which causes it to release iodine. The elemental iodine immediately combines with the SO2 to form iodide ions. The iodide-rich solution then gets a shot of iodic acid (HIO3), which converts all the iodide ions into solid iodine.
Kerosene is then added to the solution to extract the iodine. This dark purple witch’s brew is then pumped into a reactor where high temperature and pressure are used to melt the solid iodine, dropping it back out of the suspension. The molten iodine is then pumped to a prilling tower, where it drips through a sieve of fine holes. The droplets cool as they drop through the tower, forming small balls of solid iodine, or prills, which are ready for shipping.
What's Your Reaction?
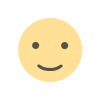
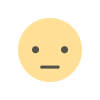
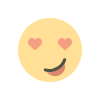
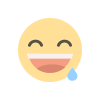
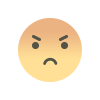
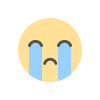
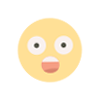